Baptiste Auguié
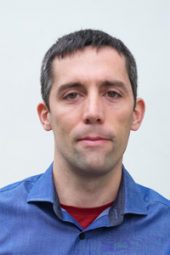
2016: Dr Baptiste Auguié, Victoria University of Wellington, School of Chemical and Physical Sciences, has been awarded a Rutherford Discovery Fellowship for research entitled: 'Light and chirality at the nanoscale'.
Biography
Dr Baptiste Auguié is a physicist specialised in nano-optics, the interaction of light with nano-structured materials. He studied physics and engineering in France and Canada, before undertaking a PhD in 2005 with Prof William Barnes at the University of Exeter, UK, with a thesis focused on the optical properties of gold nanoparticles. Noble metals such as gold and silver can be engineered to exhibit unique optical characteristics with enhanced light-matter interaction that finds potential applications in ultra-sensitive molecular detection and novel optical functions. A passion for this subject motivated him in 2010 to join the colloidal synthesis group of Prof Luis Liz-Marzán in Spain, where he participated in the pioneering exploration of metal nanoparticles assembled into helical structures. He first came to New Zealand in 2013 to join the Raman spectroscopy group of late Prof Pablo Etchegoin and Prof Eric Le Ru at Victoria University in Wellington, where he investigated optical spectroscopy techniques and original approaches for the theoretical modelling of light scattering. After another post-doctoral position with Prof Alejandro Fainstein in San Carlos de Bariloche, Argentina, he came back to Wellington in 2015 to become a New Zealand resident and pursue novel ideas at the intersection of nano-optics and spectroscopy, with post-doctoral positions at the University of Wellington, and at the Photon Factory in Auckland.
Research summary
A cursory glance at our shoes, or an awkward hand-shake with someone offering us their left hand, vividly illustrate a fundamental property of nature: chirality. Chirality refers to the characteristic dissymmetry of objects that cannot be superimposed with their mirror-image, such as our hands, feet, or the helix of DNA. Even though chiral molecules of opposite handedness are made of the same atoms, share the same physical and chemical properties, their interaction with other chiral entities can be dramatically different. Much like trying to fit a left foot into the right shoe, chiral molecules generally interact and assemble into larger structures only if they have compatible handedness. As such, chirality is central to our understanding of life at the molecular level, and permeates all length scales, from smaller molecules to DNA, viruses and bacteria, all the way to macroscopic patterns of more familiar experience such as sea shells or ferns. Likewise, chirality is key to the design of pharmaceuticals, as many drugs require a specific molecular handedness to have the desired effect on living bodies; their chiral alter ego might be inactive, or worse, a poison.
Ever since its discovery, our understanding of chirality has relied heavily on optical methods for detection and characterisation. Light interacts with chiral materials in subtle ways – some of which remain largely unexplored or poorly understood –, and the chiral signature is generally very weak, requiring large amounts of material to detect a specific handedness. This limitation to bulk samples has hindered the development of chiro-optical methods toward ultra-sensitive detection.
To overcome the intrinsic weakness of chiral interactions between light and molecules, Dr Auguié proposes to harness novel artificial structures comprising metallic nanoparticles. Metals such as gold and silver scatter light very efficiently, and can enhance light-matter interactions at the nanoscale by many orders of magnitude. This project will explore chiral spectroscopies of molecule–metal nanoparticle assemblies, where the light scattered by such chiral samples will be analysed as a function of both wavelength and optical rotation. Such techniques will allow unprecedented access to chirality at the nano and molecular scales, with strongly enhanced optical signals. These fundamental advances will guide the design of novel sensors with great potential for analytical applications, particularly in the life sciences.